The various testing protocols (Brinell, Rockwell, Vickers) have procedures that are specific to the item under test. The Rockwell T test is adapted to check light-wall tubing by cutting the tube lengthwise and testing the wall from the ID rather than the OD.
Ordering tubing is a little like going to an auto dealership and ordering a car or a truck. The many options available these days allow the buyer to customize the vehicle in all sorts of ways—interior and exterior colors, interior trim packages, exterior styling options, drivetrain choices, and sound systems that nearly rival home entertainment systems. Given all these choices, you probably wouldn’t settle for a standard, no-frills vehicle. 2x2 Square Tubing
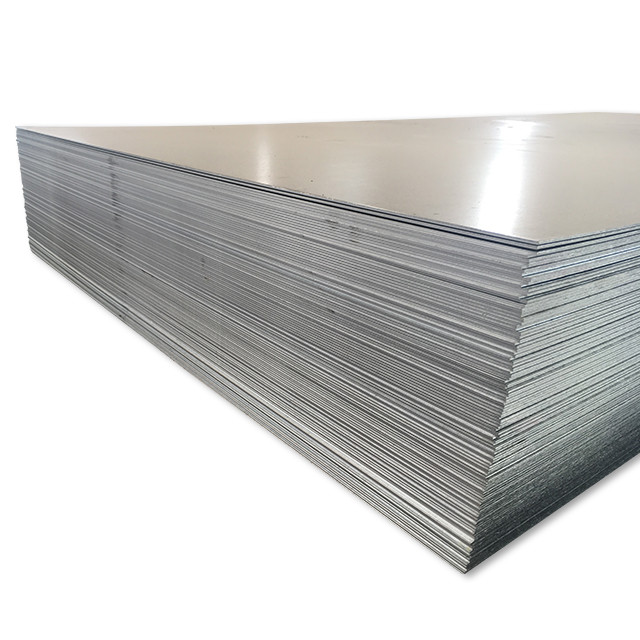
Steel tubing is like that. It’s available in thousands of choices, or specifications. Dimensions aside, the specifications call out the chemistry and several mechanical properties, such as minimum yield strength (MYS), ultimate tensile strength (UTS), and minimum elongation before failure. However, many in the industry—engineers, purchasing agents, and fabricators—use an accepted industry shorthand and ask for a “plain” welded tube and specify just one characteristic: hardness.
Try ordering a car by a single characteristic (“I need one with automatic transmission”) and you won’t get too far with the salesman. He has to fill out an order form, and it has many options on it. Steel tubing is like that—to get the right tube for the application, the tube manufacturer needs much more information than just the hardness.
How did hardness become an accepted substitute for the other mechanical properties? It may have started with tube producers. Because a hardness test is quick, easy, and requires relatively inexpensive equipment, tube salesmen often used a hardness test to compare two tubes. To perform hardness test, they just needed a smooth section of tube and a test stand.
A tube’s hardness correlates well with the UTS, and from there a rule of thumb, a percentage or range of percentages, helps to estimate the MYS, so it’s easy to see how the hardness test caught on as a suitable proxy for other characteristics.
Also, the other tests are relatively complicated. While a hardness test takes just a minute or so on one machine, the MYS, UTS, and elongation tests require sample preparation and a substantial investment in large-scale laboratory equipment. As a comparison, think in terms of seconds for a hardness test by a tube mill operator and hours for a tensile test by a dedicated metallurgy technician. Making a hardness check is not hard.
This isn’t to say that engineered tube manufacturers don’t use hardness testing. It’s safe to say that most do, but because they perform gauge repeatability and reproducibility evaluations on all of their test equipment, they are well aware of the test’s limitations. Most use evaluate a tube’s hardness as part of the production process, but they don’t use it to quantify the tube’s characteristics. It’s merely a go/no-go test.
Why do you need to know the MYS, UTS, and minimum elongation? They indicate how the tube will perform in an assembly.
The MYS refers to the lowest amount of force that causes a permanent deformation in a material. If you try to bend a straight length of wire such as a coat hanger just a bit and release the pressure, one of two things will happen: It will spring back to its original condition (straight) or it will remain bent. If it’s still straight, you haven’t exceeded the MYS. If it remains bent, you have exceeded it.
Now, grab both ends of the wire with sets of pliers. If you can tear the wire into two pieces, you have exceeded its UTS. You put a lot of tension on it and you have two lengths of wire to show for your superhuman effort. If the original length of wire was 5 inches, and the two lengths after failure add up to 6 in., the wire elongated 1 in., or 20 percent. An actual elongation test takes a measurement within 2 in. of the failure point, but no matter—the wire pull concept illustrates UTS.
Steel micrograph samples require cutting, polishing, and etching with a mildly acidic solution, usually nitric acid and alcohol (nital), to make the grains visible. Magnification at 100 power is common for examining steel grains and determining the grain size.
Hardness is a test of how the material responds to an impact. Imagine putting a short length of tube into a vise, one with serrated jaws, and cranking the vise closed. In addition to flattening the tube, the vise’s jaws leave impressions on the tube’s surface.
Hardness testing works like that, but it’s not as crude. The test has a controlled size of impact and controlled pressure. These forces deform the surface, making an indentation or an impression. The size or depth of the impression determines the metals’ hardness.
For evaluating steel, common hardness tests are Brinell, Vickers, and Rockwell. Each has its own scale, and some have a variety of test methods, such as Rockwell A, B, and C. For steel tubing, ASTM specification A513 cites the Rockwell B test (abbreviated HRB or RB). The Rockwell B test measures the difference in penetration into the steel by a 1⁄16-in.-diameter steel ball between a minor applied preload and a major load of 100 kilograms of force. A typical result is HRB 60 for standard, low-carbon steel.
Material scientists know that hardness has a linear relationship to UTS. Therefore, a given hardness predicts the UTS. Likewise, tube producers know that the MYS and UTS are related. For as-welded tubing the MYS usually is from 70 to 85 percent of the UTS. The exact amount depends on the process used to make the tube. A hardness of HRB 60 correlates to a UTS of 60,000 pounds per square inch (PSI) and an MYS around 80 percent of that, or 48,000 PSI.
The most common tubing specification for general fabrication is maximum hardness. Aside from the dimensions, engineers are concerned with specifying an as-welded electric resistance welder (ERW) tube within a good working range, and that can result in a maximum hardness of perhaps HRB 60 finding its way onto the component part drawing. This decision alone leads to a range of resulting mechanical properties, including hardness itself.
First, a hardness of HRB 60 doesn’t really tell us much. The reading, HRB 60, is a dimensionless number. A material evaluated at HRB 59 is softer than a material tested at HRB 60, and HRB 61 is harder than HRB 60, but by how much? It’s not quantifiable like a volume (measured in decibels), torque (measured in pound-feet), speed (measured as a distance relative to time), or UTS (measure in pounds per square inch). The reading, HRB 60, doesn’t tell us anything specific. It is a characteristic of the material, but it is not a physical property. Second, hardness testing doesn’t lend itself well to repeatability or reproducibility. Evaluating two locations on a test specimen, even when the test locations are near each other, often results in hardness readings that vary significantly. Compounding this problem is the nature of the test. After a location is measured, it cannot be measured a second time to verify the result. Repeatability of test is impossible.
This doesn’t mean that hardness testing isn’t handy. Indeed, it provides a good guide to the material’s UTS, and it’s a quick and easy test to perform. However, everyone involved in specifying, purchasing, and fabricating tube should be aware of its limitations as a test parameter.
Because “plain” tube isn’t defined very well, when it is requested, the tube manufacturer often narrows this down to two of the most commonly made steel and steel tube types defined in ASTM A513: 1008 and 1010. Even after eliminating all other tube types, the possibilities in mechanical properties in these two tube types are wide open. Indeed, these tube types have the widest range of mechanical properties of any types of tubing.
For example, if the MYS is low and the elongation is high, the tube is described as soft, meaning it will perform better in stretching, deflection, and permanent distortion than a tube described as hard, which has a relatively high MYS and relatively low elongation. This is similar to the difference between a soft wire and a hard wire, such as a coat hanger and a drill bit.
Elongation by itself is another matter that makes a big difference in critical tube applications. A tube with high elongation is forgiving of tensile forces; one with low elongation is more brittle and therefore more prone to catastrophic, fatigue-type failure. However, elongation is not directly related to the UTS, which is the only mechanical property that correlates directly to hardness.
Why do the tube mechanical properties vary so much? First, the chemistries vary. Steel is a solid solution of iron and carbon, plus other significant alloys. For simplicity we will deal only with carbon percentage here. The atoms of carbon replace some of the iron atoms, creating a steel crystalline structure. ASTM 1008 is the catch-all, beginning grade, and has from 0 to 0.10 percent carbon. Zero is a very special number, and it creates unique properties when carbon is ultralow in steel. ASTM 1010 has a defined carbon content between 0.08 and 0.13 percent carbon. These differences don’t appear to be large, but they are large enough to make big differences elsewhere.
Second, steel tubing can be made, or made and subsequently processed, in seven distinct manufacturing processes. ASTM A513, which pertains to ERW tube production, calls out seven types:
1b. Hot-rolled, pickled, and oiled
The Devil Is in the Details
If the steel chemistry and the tube manufacturing steps don’t make all the difference on the steel’s hardness, what does? Answering that question means taking a close look at the details. That question leads to two more questions: Which details, and how close?
The details concerning the grains that make up the steel is the first answer. When the steel is made at the primary steel mill, it doesn’t cool to become a vast mass with a single set of characteristics. As the steel cools, the molecules of steel become organized in repeated patterns (crystals), similar to the way snowflakes form. After the crystals form, they aggregate into groups called grains. As the cooling progresses, the grains grow, forming throughout the sheet or plate. As the last of the steel molecules get absorbed by the grains, the grains stop growing. All of this happens at the microscopic level, in that an average size steel grain is about 64 µ or 0.0025 in. wide. While each grain is similar to the next, they aren’t identical. They vary slightly from one to the next in size, orientation, and carbon content. The interfaces between grains are called grain boundaries. When steel fails, for example by a fatigue crack, it tends to fail along the grain boundaries.
How close do you have to look to see discernible grains? A magnification of 100 power, or 100 times as acute as human vision, is plenty. However, merely looking at unprepared steel at 100 power wouldn’t reveal much. Samples are prepared by polishing them and etching the surface with an acid, typically nitric acid and alcohol called a nital etch.
It is the grains and their internal crystalline lattice that determine the impact strength, MYS, UTS, and the extent of elongation the steel can withstand before failure.
Steelmaking steps, such as hot rolling and cold rolling of strip, impart stresses into the grain structure; if they permanently change the shape, this means that the stresses have deformed the grains. Other processing steps, such as rolling the steel into a coil, uncoiling it, and running it through a tube mill (forming the tube and sizing it) deform the steel grains. Cold drawing a tube over a mandrel also stresses the material, as do fabrication steps such as end forming and bending. A change in the grain structure is called a dislocation.
The aforementioned steps can use up the steel’s ductility, which is its ability to withstand tensile (pull-apart) stress. The steel becomes brittle, meaning it is more likely to fracture if you continue to work the steel. Elongation is one component of ductility (compressibility is another). Here it is important to understand that failures most often happen during tensional stress, not compression. Steel is pretty strong against tensile stress, as it has a relatively high elongation capability. However steel under compressive stress deforms easily—it is malleable—and that’s an advantage.
Compare this to concrete, which has very high compressive strength but low ductility. These characteristics are the opposite of steel’s. This is why concrete used in roads, buildings, and sidewalks commonly is installed with steel reinforcing bar. The result is a product that has the benefits of both materials’ strengths: Under tension the steel is strong, and under compression the concrete is strong.
During cold working, as the steel’s ductility drops, its hardness increases. In other words, it work-hardens. Depending on the specifics of the situation, this can be a benefit; however, it can be a drawback in that hardness equates to brittleness. That is, as the steel becomes harder, it becomes less elastic; it therefore is more likely to fail.
In other words, each process’s step uses up some of the tube’s ductility. As the part has been worked, it has become progressively harder, and if it’s too hard, it’s basically useless. Hardness is brittleness, and a brittle tube is likely to fail when used.
In such a case, does the fabricator have any options? In short, yes. That option is annealing, and while it’s not quite magic, it’s about as close to magic as you can get.
In layman’s terms, annealing undoes everything that physical stresses do to metals. The process heats the metal to a stress relief or recrystallization temperature, which eradicates the dislocations. The process thereby restores some of its ductility, or all of the ductility, depending on the specific temperature and time used in the annealing process.
Annealing and controlled cooling promote grain growth. This is beneficial if the goal is to reduce the material’s brittleness, but uncontrolled grain growth can soften the metal too much, making it unusable for its intended application. Stopping the annealing process is another bit of near-magic. A quench, done at the right time, with the right quenchant, at the right temperature, brings the process to a rapid halt, capturing the steel’s restored properties.
Should we dispense of the hardness specification? No. When specifying steel tubing, hardness properties are valuable mainly as a reference point. A useful measurement, hardness is one of several characteristics that should be specified when ordering tubular material and checked when receiving a shipment (and it should be recorded for every shipment). When a hardness check is an inspection criterion, it should have the proper scale value and control limits.
However, it’s not a true test used for qualifying (accepting or rejecting) material. In addition to hardness, fabricators should occasionally have shipments tested to determine the other relevant characteristics, such as MYS, UTS, or minimum elongation, depending on the tube’s application.
Wynn H. Kearns handles regional sales for Indiana Tube Corp., 2100 Lexington Road, Evansville, IN 47720, 812-424-9028, wkearns@indianatube.com, www.indianatube.com.
See More by Wynn H. Kearns
Read more from this issue
Subscribe to The Tube & Pipe Journal
The Tube & Pipe Journal became the first magazine dedicated to serving the metal tube and pipe industry in 1990. Today, it remains the only North American publication devoted to this industry, and it has become the most trusted source of information for tube and pipe professionals.
Easily access valuable industry resources now with full access to the digital edition of The Fabricator.
Easily access valuable industry resources now with full access to the digital edition of The Welder.
Easily access valuable industry resources now with full access to the digital edition of The Tube & Pipe Journal.
Easily access valuable industry resources now with full access to the digital edition of The Fabricator en Español.
In this episode of The Fabricator Podcast, and in partnership with Miller Electric, Andy Weyenberg from Miller's motorsports...
© 2024 FMA Communications, Inc. All rights reserved.

Steel Plate Not yet registered? Sign up